Basic Cell Biology
What is a Cell?
Mammalian cells are enclosed by a plasma membrane, which consists of a lipid bilayer. The space enclosed by the plasma membrane is called the cytoplasm. Organelles, which are integral to the functioning of the cell, are found in the cytoplasm. Organelles include:
Nucleus The nucleus contains most of the cell’s DNA molecules organised into structures called chromosomes. To activate genes, proteins need to enter the nucleus via pores.
Endoplasmic Reticulum There are two types of ER: rough endoplasmic reticulum (RER) and smooth endoplasmic reticulum (SER). RER is studded with ribosomes, while SER lacks ribosomes. Ribosomes are complex molecular machines that are important for protein synthesis and can be found both bound to RER and free in the cytoplasm.
Golgi Apparatus The Golgi is a series of flattened membrane-bound sac where proteins made in the ER are modified, sorted and packaged for transport to their eventual destinations within the cell.
Mitochondria The mitochondrion is enclosed in a double membrane: an inner membrane and an outer membrane. The mitochondrion is often described as the ‘powerhouse’ of the cell because it generates most of the cell’s supply of energy.
Lysosome The lysosome is a membrane-bound sac containing enzymes, which digests proteins and other cellular byproducts.
—
Kevin Mackenzie, University of Aberdeen, Wellcome Images / CC BY 4.0
Above is an image of a human liver cell taken with an electron microscope. Can you identify all the numbered organelles? (Please respond in lowercase to ensure that your answers validate correctly.)
1.
2.
3.
4.
HINT We haven't labelled any endoplasmic reticulum.
DNA & the Central Dogma
All of the instructions that a cell needs to produce its proteins are encoded in its DNA (deoxyribonucleic acid). A DNA molecule is a double stranded α-helix, consisting of a backbone made up of phosphate and deoxyribose sugars, with nitrogenous bases acting like rungs of a ladder, connecting the two strands. There are four bases - guanine (G), cytosine (C), adenine (A), and thymine (T). G and C are complementary to each other, as are A and T, meaning that their structure allows them to fit nicely together, like a lock and key. Hydrogen bonds link these complementary bases together, thereby connecting the two DNA strands. A phosphate group connected to deoxyribose sugar and a nitrogenous base is known as a nucleotide.
The total length of DNA in a single cell is approximately 2 metres! How does this all fit inside such a tiny cell? Well, to achieve this, DNA is wrapped around proteins, called histones. It is then coiled into a structure called a chromosome. In the nucleus of all human cells, there are 23 pairs of chromosomes, 22 pairs of autosomes and one pair of sex chromosomes. Our chromosomes and genes are highly regulated and well balanced like a old recipe, any deviation in the amount of chromosomes or genes are generally not well tolerated and can be the basis of cancer development. [See Genetic Basis of Cancer]
Did you know that humans share about 50% of the same genome as the fruit fly? It is not too surprising given that all organisms share a common ancestor who lived a billion years ago. Although we may share a conserved genome, the difference is in the expression of our genes.
Each DNA molecule can be divided into millions of smaller segments called genes. Humans have an estimated 19,000-20,000 genes. The size of a gene can vary from as few as 100 base pairs to as many as 80,000 base pairs. Each gene contains the instructions needed for the production of precisely one protein. The process by which a cell uses the instructions encoded by a gene to produce a protein is described in the central dogma of molecular biology, which was first proposed by Francis Crick, co-recipient of the 1962 Nobel Prize for Physiology or Medicine.
Transcription
When a cell is ready to produce a protein, an enzyme called RNA polymerase II comes along and unwinds the DNA molecule at the position of the gene that encodes that protein. RNA polymerase II then uses one of the DNA strands as a template to synthesise a messenger RNA (mRNA) molecule in a process called transcription. The mRNA molecule is then processed: a 7-methylguanylate cap is added to the start of the molecule, sections of the molecule are spliced out, and a poly(A) tail is added to the end of the molecule. After RNA processing, mRNA molecule is then exported out of the nucleus into the cytoplasm.
Translation
In the cytoplasm, a ribosome comes along and binds to the mRNA molecule. The ribosome recruits transfer RNA (tRNA) molecules carrying specific amino acids to the mRNA molecule, and then joins the amino acids together to form the protein in a process called translation. The newly synthesised protein then wanders off to perform its specific function. Hence, the central dogma can be distilled to the following statement: genetic information flows from DNA to RNA to protein.
Which of the following statements about DNA and the Central Dogma is correct?
Cell Cycle
The cell cycle refers to the sequence of events that cells go through to divide and replicate. It consists of a number of phases:
State | Phase | Code | Features |
---|---|---|---|
Resting | Quiescence | G0 | Some cells leave the cell cycle and enter a 'resting' phase call the G0 phase. Such cells are called senescent cells. Examples of senescent cells include neurons and cardiac muscle cells. |
Interphase | Growth 1 | G1 | The cell grows in size. The G1 checkpoint ensures that the cell is ready for DNA synthesis. |
Interphase | Synthesis | S | The cell replicates its DNA. After S phase, the amount of DNA in the cell doubles. |
Interphase | Growth 2 | G2 | The cell continues to grow. The G2 checkpoint ensures that cell is ready for mitosis. |
Dividing | Mitosis | M | The cell stops growing. The cell passes through a number of phases (prophase, metaphase, anaphase, telophase and cytokinesis), at the end of which the cell divides into two genetically identical daughter cells. |
The cell cycle is very important in growth and repair of tissues. Cells of different tissues go through the cycle at different rates which depend largely on microenvironment of the cell and its intrinsic nature. Some cells like epithelial skin cells are constantly entering the cell cycle because they are continually under stress from the environment, other cells such as liver cells are usually resting but will enter the cell cycle when there is injury or damage and the rest are permanent cells meaning they have no ability to divide. Typical cancer cells are like epithelial cells in that they are constantly dividing at a fast rate.
Check out this interesting short video showing cell mitosis in action!
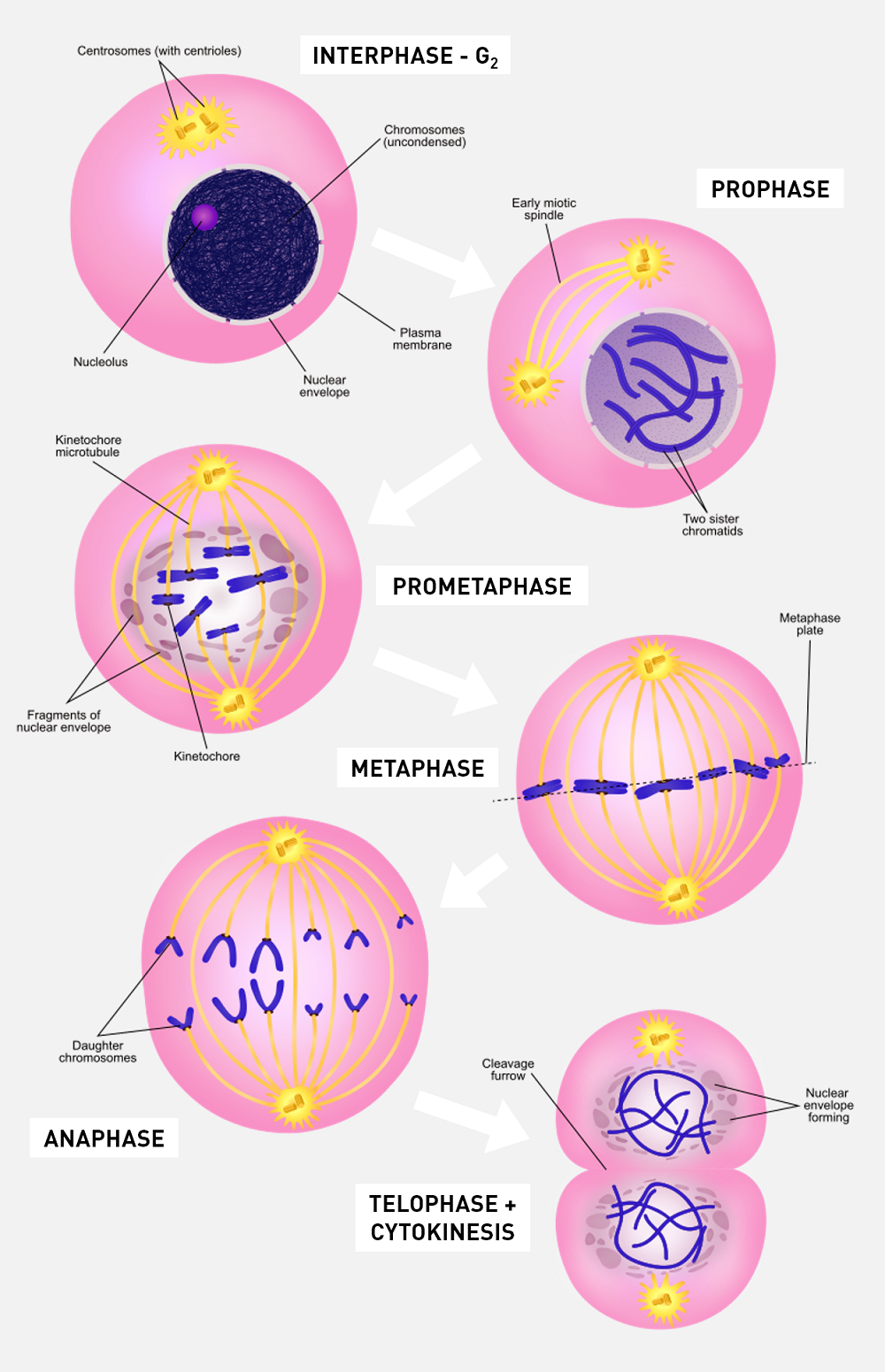
Which of the following statements about the cell cycle is correct?
Cell Signalling
If cells enter the cell cycle and duplicate themselves whenever they want, the body may end up with too many cells, more than what it needs. If the body doesn’t put a stop to this uncontrolled growth, it may end up with a tumour. So it’s really important to regulate where and when a cell enters the cell cycle.
How does a cell know when to enter the cell cycle? It needs to be told what to do somehow. This is where cell signalling comes in.
When a cell is required to divide and replicate, three things happen:
- The cell receives a specific signal to start the cell cycle
- The message is passed on inside the cell to the nucleus
- The cell responds to the message and enters the cell cycle
Now let’s look at each stage in more detail.
1. The cell receives a specific signal to start the cell cycle
- A signalling molecule (in this case a growth factor) binds to a receptor (growth factor receptor) on the outside of the cell.
- The receptor usually has 3 domains:
- an extracellular domain (outside the cell)
- a transmembrane domain (straddles the lipid bilayer)
- a cytoplasmic domain (inside the cell)
- The growth factor binds to the extracellular domain of the growth factor receptor in a lock-and-key fashion .
2. The message is passed on inside the cell to the nucleus
- Binding of the growth factor to the extracellular domain of the growth factor receptor causes the cytoplasmic domain of the growth factor receptor to undergo some change.
- This change is detected by special proteins inside the cytoplasm of the cell called kinases.
- These kinases then initiate a cascade of reactions inside the cell that typically ends with a transcription factor moving from the cytoplasm into the nucleus.
3. The cell responds to the message and enters the cell cycle
- The once transcription factors enter the nucleus, they start to activate certain genes.
- These genes are required for progression through the cell cycle.
- The cell thus enters the cell cycle.
To give you a taste of how long and convoluted these signalling pathways can be, here is an overview of one of the most well-known: the Ras-Raf-Mek-Erk pathway, which is affectionately abbreviated to Ras.
- The Ras-Raf-Mek-Erk signalling pathway begins with a growth factor binding to a growth factor receptor.
- Binding of growth factor to the receptor causes the receptor to activate itself.
- GRB2 is a protein inside the cell that can recognise when the receptor is activated.
- GRB2 binds to the activated receptor.
- SOS is another type of protein that binds to the GRB2-receptor complex.
- After SOS docks onto GRB2, it activates another protein called Ras.
- Ras then activates a protein called Raf.
- Raf then activates a protein called Mek.
- Mek then activates a protein called Erk.
- Erk then activates a transcription factor, which moves from the cytoplasm into the nucleus to activate expression of Myc, which is itself a transcription factor.
- After Myc is produced, Myc remains in the nucleus to activate expression of Cyclin D.
- Cyclin D then forms a complex with CDK4.
- The Cyclin D/CDK4 complex then activates a protein called Rb, which is usually bound to another protein called E2F.
- Activation of Rb results in letting go of E2F.
- E2F is now free to activate expression of Cyclin E and Cyclin A, two genes that push the cell from G1 phase to S phase.
When things go wrong
It is a breakdown in the signalling pathways that control how a cell grows, divides and dies that can cause it to become cancerous. We'll talk more about this in the Hallmarks of Cancer section.
For example, problems with the Ras pathway can cause cancers of the eye or cervix.
Retinal Cancer In humans, the gene that encodes Rb is located on the long arm of chromosome 13. If both copies of the Rb gene is mutated in retinal cells, then the cell loses the ability to produce Rb. As a result, E2F becomes free to drive progression from G1 phase to S phase even in the absence of growth factor signalling. Retinal cells divide and replicate in an unregulated fashion, leading to the development of retinoblastoma, which is the most common malignant cancer of the eye in children. Although most children survive this cancer, some may lose vision in the affected eye, or need to have the eye removed.
Cervical Cancer The human papillomavirus (HPV), after it infects the keratinocytes of the cervix, produces a protein called E7, which binds to Rb, preventing E2F from binding. As a result, E2F, again, becomes free to drive progression from G1 to S phase even in the absence of growth factor signalling. Keratinocytes divide and replicate in an unregulated fashion, leading to the development of cervical cancer, which is the fourth most common cancer in women.
Types of Tissues
A group of cells with similar structure and function are organised into tissues. The four main types of tissue are epithelial tissue, connective tissue, muscular tissue and nervous tissue. Tissues are then arranged into organs.
Tissue | Features |
---|---|
Epithelial | Lines body surfaces, including skin and the lining of the oesophagus and lungs. |
Connective | Includes blood, dermis, bone and lymph. Lymph tissue makes up a large part of the immune system and helps to transport white blood cells to and from the bloodstream. |
Muscular | Includes skeletal (voluntary), smooth (involuntary e.g. oesophagus) and cardiac (involuntary) muscle. |
Nervous | Comprises neurons. |
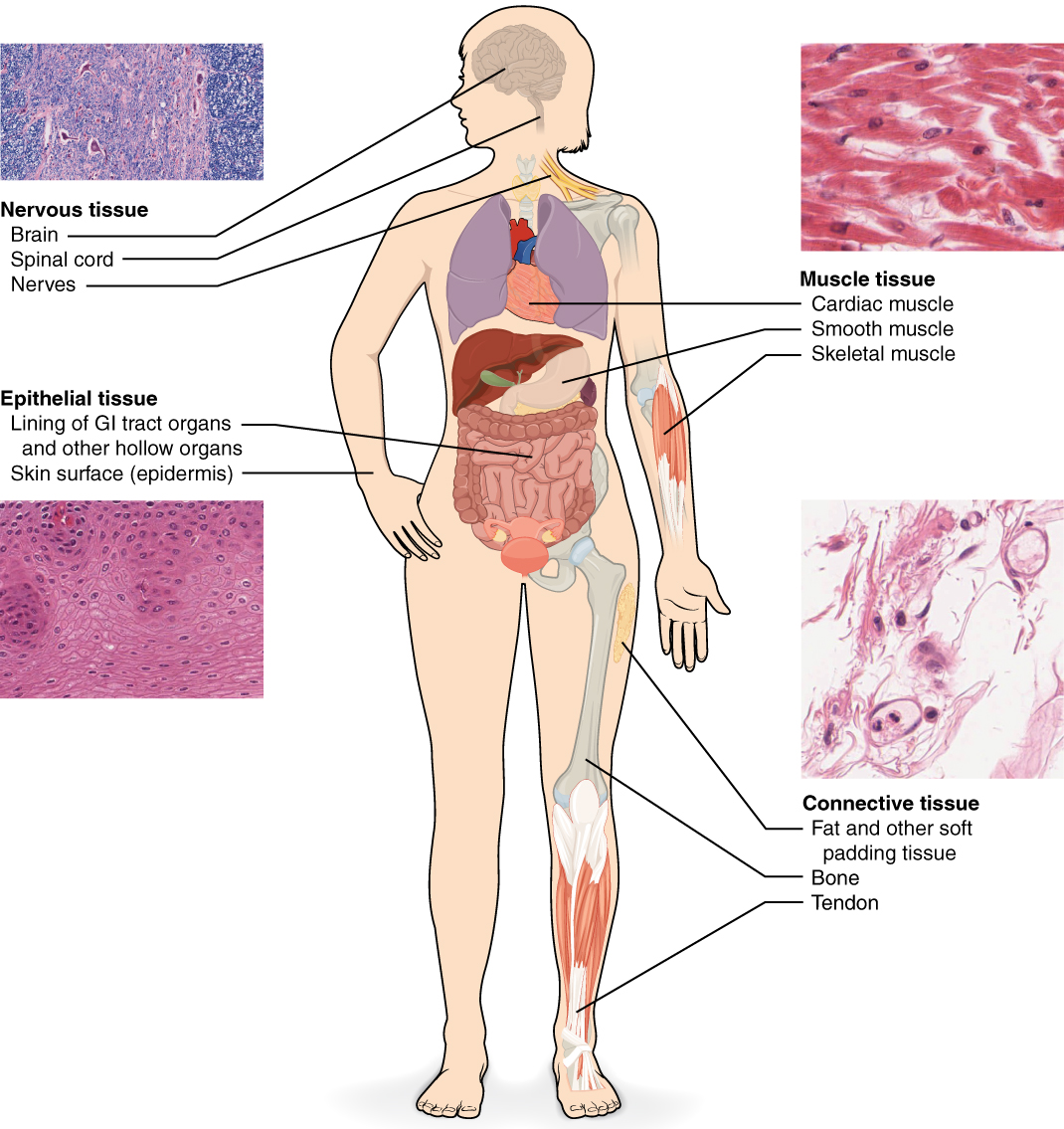
Cells in each type of tissue are arranged and connected to each other differently. This allows tissues to have different characteristics and functions. For example, epithelial cells are intimately connected with their neighbours by different types of cell junctions. A layer of epithelial cells is also anchored to a thin meshwork of connective tissue, known as the basement membrane. These connections are very strong, and are the reason why skin is resistant to stretching and other stresses. As a consequence, epithelial cells have a specific orientation; one side must be connected to the basement membrane, and others must be linked to adjacent epithelial cells. On the other hand, some cells in connective tissues (e.g. red blood cells) are not connected to their neighbours and are therefore more mobile. This means that abnormalities can arise when tissues lose their characteristics. For example, if cells in epithelial tissues lose their cell junctions, they can become detached from the basement membrane and might enter the bloodstream. The migration of epithelial cells and the loss of epithelial characteristics are known as epithelial-mesenchymal transition (EMT). EMT is a key step in many cancers and is the reason to how cancers are spread in the body.
Which of the following statements about tissues is correct?